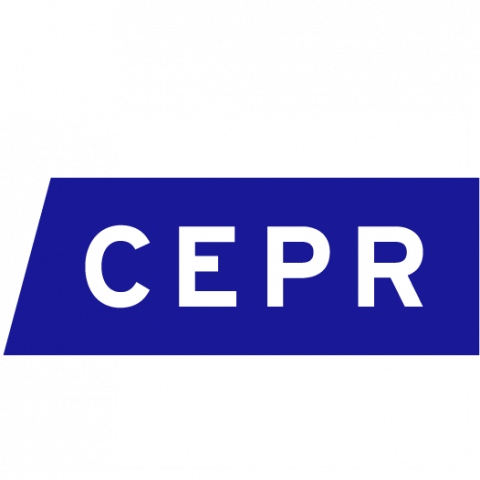
Anthropogenic climate change will likely intensify the negative environmental impacts of agriculture through powerful feedback loops. This has important implications for development research, policy and R&D investment.
Agriculture has large global environmental impacts, from greenhouse gas emissions to water and air pollution to biodiversity loss (Campbell et al. 2017). Analysts have long emphasised that rising demand for agricultural products, primarily driven by population and income growth in low- and middle-income countries (LMICs), threatens to expand agriculture’s climate and environmental footprint due to the increased derived demand for land, water and agrochemicals (Tilman et al. 2011, Williams et al. 2021). At the same time, an expanding scientific literature has shown how anthropogenic climate and environmental changes impact agricultural production because crop and livestock systems depend fundamentally on growing conditions. The potential for positive (i.e. self-reinforcing) feedback within this closely coupled system has attracted far less attention, despite its powerful implications.
In a new review paper in Science, Yang et al. (2024) synthesise the scientific evidence around the multiple pathways through which anthropogenic climate change is likely to intensify agriculture’s negative environmental impacts. The review highlights potentially powerful feedback loops that amplify greenhouse gas emissions through increasing CH4 emissions from rice paddies, N2O emissions from soil, and CO2 emissions from land clearing and soil tillage.
Beyond greenhouse gas emissions, agriculture’s environmental impacts per unit output will likely increase. Climate change, on average, increases water stress, soil erosion, nitrogen and phosphorus pollution, and biodiversity loss. It also dampens agricultural productivity growth, reduces the efficacy of agrochemicals, and alters and sometimes accelerates the spread of crop diseases and pests. These effects could indirectly drive further land clearing, especially in sub-Saharan Africa and South and Southeast Asia, where future demand for agricultural products is concentrated and thus where most supply expansion must occur. Figure 1, reproduced from Yang et al. (2024), illustrates these feedback channels.
Figure 1
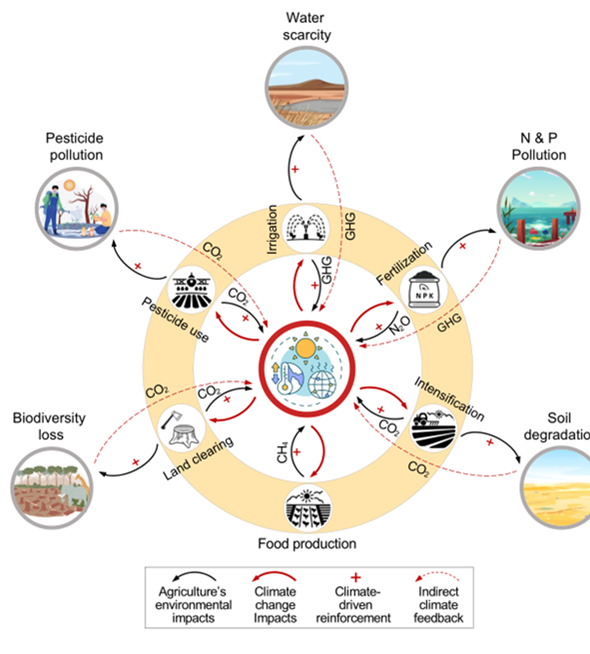
Notes: The major environmental impacts of agricultural systems and potentially exacerbating effects of climate change. The dark red circle in the centre represents climate change. The yellow donut represents agricultural systems and small circles inside indicate the processes through which it affects the environment (black arrows). Dark red arrows indicate impacts of climate change on agricultural, and plus signs indicate its reinforcing effects on agriculture's environmental problems, including direct feedback to climate change (e.g. intensified CH4 and N20 emissions). Dashed arrows indicate indirect climate-change feedback from the different environmental impacts of agriculture (e.g. stimulated CH4 emissions in lakes from nutrient runoff). Figure from Yang et al. (2024).
Why is it important to understand these feedback mechanisms between climate change and agriculture?
In short, we have likely underestimated the challenge of sustainably feeding the world and achieving inclusive economic growth in the 21st century by not accounting for these pernicious cycles. Previous work (e.g. Barrett et al. 2023) documents the interplay among agriculture, climate change and the natural environment and its profound implications for factor productivity, economic growth, and the structural transformation of LMICs. Agriculture and economic development do not operate in isolation from the natural environment; they are inextricably linked.
Economic activities, including agricultural production, directly impact natural systems, often leading to environmental changes that alter the relative returns to different activities and technologies, generating feedback that shapes economic growth and development processes. The new analysis in Yang et al. (2024) draws out key dimensions of the challenge in new detail that is directly useful for economists and development practitioners, who have been slow to recognise and act on the necessity of transitioning agrifood systems to achieve health, equity, resilience and sustainability objectives, like those enshrined in the Sustainable Development Goals (Barrett et al. 2023).
Evidence on sustainable and productive agricultural practices
Many existing agricultural practices and technologies have been rigorously shown to simultaneously improve agricultural sustainability, productivity, and agricultural systems’ resilience to climate change’s negative impacts, at least under certain conditions. Chief among these are:
- Expanded use of improved cultivars that increase food output per unit of land, water, and chemical input, often through genetic resistance to pests and pathogens.
- Integrated pest management that boosts crop output while minimising the use of toxic chemicals.
- Integrated soil fertility management that combines biological, chemical, and physical measures to sustain soil health.
- Agricultural diversification that improves yield and yield stability, resource use efficiency, biodiversity, and human well-being (Jain et al. 2023).
Crop genetic innovations have had sizeable impacts on economic and population growth in LMICs with widespread adoption (Gollin et al. 2021).
Implications for development research
The reinforcing feedback between climate change, agricultural production and environmental degradation that Yang et al. (2024) highlight raises the expected returns to, and the urgency of, investments in context-specific research aimed at identifying and overcoming socioeconomic barriers to adoption of sustainable agricultural practices and technologies.
Climate change adaptation for LMICs also requires adjusting policies and R&D investment patterns which have historically focused on staple cereals and tubers. Further, incentives need to shift to diversifying production to respond to market demand and to address the environmental externalities associated with staple crops and feedgrains (FAO 2023).
More development research should focus on bundled socio-technical interventions to inform potential complementarities, because past successes – including the Green Revolution successes (Gollin et al. 2021) or China’s Science and Technology Backyard program (Zhang et al. 2016; An et al. 2024) – have arisen from context-appropriate combinations of technological, policy and institutional innovations (Barrett et al. 2023). The rising urgency of research to identify context-specific solutions likewise favours studies designed as A/B trials among alternatives rather than as control/treatment trials that intrinsically assume that doing nothing is a viable policy option.
Greater investment is also needed in forward-looking R&D to develop new foods and methods of producing familiar ones. The findings of Yang et al. (2024) suggest that the returns are especially high to:
- The discovery, adaptation and cost reduction of emerging agricultural technologies that make agriculture more productive, sustainable, and climate resilient.
- The development of cost-competitive novel foods whose production requires far less land, water, and chemicals per capita than do prevailing agricultural practices.
Bidirectional linkages between economic activity and the climate
Development scholars and practitioners must embrace the possibility and challenge of “green rural transformation” (Barbier 2020), the need for which grows increasingly urgent as science sheds greater light on the reinforcing feedback between agriculture, climate change, and environmental degradation. Effective economic development policies and associated R&D investment strategies must take seriously the bidirectional linkages among economic activity, climate, and the natural environment, especially in regions with predominantly agrarian economies. These are the locations where population and economic growth pressures on the planet loom largest and thus where the need for agricultural supply expansion is most urgent. But the challenge transcends those locations; it is planetary in scale, implicating all people and countries.
References
An, Z, Y Yang, X Yang, W Ma, W Jiang, Y Li, G Chen, W Zhang, M Zhuang, C Wang, and F Zhang (2024), “Promoting sustainable smallholder farming via multistakeholder collaboration,” Proceedings of the National Academy of Sciences, 121(21): e2319519121.
Barbier, E B (2020), “Is green rural transformation possible in developing countries?” World Development, 131: 104955.
Barrett, C B, A Ortiz-Bobea, and T Pham (2023), “Structural transformation, agriculture, climate, and the environment,” Review of Environmental Economics and Policy, 17(2): 195–216.
Barrett, C B, T Benton, J Fanzo, M Herrero, R J Nelson, E Bageant, E Buckler, K Cooper, I Culotta, S Fan, R Gandhi, S James, M Kahn, L Lawson-Lartego, J Liu, Q Marshall, D Mason-D’Croz, A Mathys, C Mathys, V Mazariegos-Anastassiou, A Miller, K Misra, A Mude, J Shen, L M Sibanda, C Song, R Steiner, P Thornton, and S Wood (2022), Socio-Technical Innovation Bundles for Agri-Food Systems Transformation, Sustainable Development Goals Series, Springer.
Campbell, B M, D J Beare, E M Bennett, J M Hall-Spencer, J S I Ingram, F Jaramillo, R Ortiz, N Ramankutty, J A Sayer, and D Shindell (2017), “Agriculture production as a major driver of the Earth system exceeding planetary boundaries,” Ecology and Society, 22: 8.
FAO (2023), The State of Food and Agriculture 2023 – Revealing the true cost of food to transform agrifood systems, Rome.
Gollin, D, C W Hansen, and A M Wingender (2021), “Two blades of grass: The impact of the green revolution,” Journal of Political Economy, 129(8): 2344–2384.
Jain, M, C B Barrett, D Solomon, and K Ghezzi-Kopel (2023), “Surveying the evidence on sustainable intensification strategies for smallholder agricultural systems,” Annual Review of Environment and Resources, 48: 347–369.
Pingali, P (2015), “Agricultural policy and nutrition outcomes – getting beyond the preoccupation with staple grains,” Food Security, 7: 583–591.
Tilman, D, C Balzer, J Hill, and B L Befort (2011), “Global food demand and the sustainable intensification of agriculture,” Proceedings of the National Academy of Sciences, 108: 20260–20264.
Williams, D R, M Clark, G M Buchanan, G F Ficetola, C Rondinini, and D Tilman (2021), “Proactive conservation to prevent habitat losses to agricultural expansion,” Nature Sustainability, 4: 314–322.
Yang, Y, D Tilman, Z Jin, P Smith, C B Barrett, Y G Zhu, J Burney, P D’Odorico, P Fantke, J Fargione, and J C Finlay (2024), “Climate change exacerbates the environmental impacts of agriculture,” Science, 385(6713): eadn3747.
Zhang, W, G Cao, X Li, H Zhang, C Wang, Q Liu, X Chen, Z Cui, J Shen, R Jiang, and G Mi (2016), “Closing yield gaps in China by empowering smallholder farmers,” Nature, 537(7622): 671–674.